Problem:
- What is the isotonic salt concentration of celery?
- How does boiling yeast cells affect active transport?
Hypothesis:
- Osmosis is a process in which solvent molecules flow and pass through a semipermeable membrane. The semipermeable membrane allows the passage of the solvent, but not the solutes. The water in an object flows through the region of a low solute concentration (hypotonic) to the higher solute concentration (hypertonic) (W1). Within a celery cell, as it is a plant cell; it contains an aqueous solution of salts, various proteins, and various enzymes. Within this experiment, it can be utilized through the observation of the celery within a considerably hypertonic and hypotonic solution in comparison to the concentration of the solution within the celery and its cells to determine the isotonic salt concentration of the celery. As celery has a considerably aqueous solution within its cells, this experiment will help to determine and prove how osmosis can affect the interactions between a hypertonic solution and a hypotonic solution, to help determine an object’s isotonic salt concentration, through its reactions to other solutions. As celery is known to behave hypotonically to salt water and hypertonic to freshwater, it can be predicted that the isotonic salt concentration of the celery will be lower to the salt water, but higher to the pure distilled water solution (W2).
- According to recent findings, fungi use vesicular transport to successfully transfer and deliver substances across their cell walls within their cell membranes. Fungi contain isolated cell walls indicating that they are semi permeable structures with an extremely limited and defined permeability (W6). Within the context of the experiment, boiling the yeast cells can damage and potentially kill the highly sensitive and complex yeast cells due to water’s high boiling temperature point. Essentially due to the yeast reaching 100 degrees celsius (the boiling point of water)(W15), it can be assumed that most if not all of the yeast cells die off, damaging it’s cell functions with it. Boiling the yeast cells not only can cause cell death, but the damage done to the cell can weaken the semipermeable membrane of the yeast cell which results in the seeping of
the congo red dye into the yeast cells which is demonstrated within this experiment through the comparison of boiled yeast cells and unboiled yeast cells.
Introduction
“Cells are the building blocks of life”, an expression that is often used when teaching biology, as it is undeniably true. Every living organism is composed of billions—if not trillions—of cells that all function together to complete the organism’s necessary tasks. A particularly significant organelle is the cell membrane.
There are three ways to classify the permeability of a membrane–impermeable, semi-permeable, and permeable. Impermeable membranes do not allow substances to pass through, semipermeable membranes are able to selectively allow certain substances to pass through, and permeable membranes allow substances to easily pass through. Substances pass through cell membranes through pathways called protein channels, or transport proteins. Transport proteins can be channel-mediated (protein opens and closes like a trapdoor) or carrier-mediated (protein rotates like a revolving door).
Figure 1: Passive vs Active Transport Visual (W9) Additionally, transport across the membrane can be either active or passive. Active transport is the process of substances moving against the concentration gradient (moving from an area of low concentration to high) and passive transport is the inverse (high concentration to low concentration), see Figure 1 for a visual representation of active and passive transport. A specific type of passive transport being observed in this lab activity through celery is osmosis.
Osmosis can be defined as the movement of water molecules across a semipermeable membrane from an area of high concentration to an area of low concentration (W14). Water enters plant cells because the overall water potential in the soil in its natural environment is higher than that of
the roots and plant parts (W12). By using specifically higher and lower concentrations ranging from 0g/100mL-2.0g/100mL, it is possible to identify how osmosis in celery cells is affected by the concentration of its environment.
Going along with the concept of active and passive transport. There are certain terms that represent the tonicity of a substance in comparison to its surroundings. The first is isotonic, meaning that the cell is in equilibrium with its surroundings, meaning the solute concentrations inside and outside of the cell are equal and therefore there is no large amounts of water movement. Next is hypotonic, meaning the solution outside the cell has a lower concentration
than inside, causing water to enter the cell. Finally, hypertonic solutions, which are solutions that have a higher concentration than inside the cell, causing water to rush out, making the cell shrivel and wrinkle (W3). In this lab activity, the isotonic salt concentration of celery will be determined by observing whether the celery expands due to water entering the cell (solution around the celery is hypotonic) or whether the celery shrinks (solution around the celery is hypertonic).
Furthermore, the cytoplasm is made up of a thick aqueous solution containing various metabolites, ions, macromolecules, and other solvents (W11). The concentration of an organism’s cytoplasm should reflect the concentration of its environment in order to maintain homeostasis. When an organism has an inner concentration that is higher than that of its environment, it will attempt to dilute its contents in order to maintain dynamic equilibrium.
By adding an excessive amount of water into the cell, the membrane will become more pressured, affecting something called the turgor pressure. Turgor pressure can be defined simply as the pressure that is exerted by the fluid against the cell wall (W10). In the opposite situation, as water exits the cell to match an environment of higher concentration, the turgor pressure will then decrease. The process of water exiting the cell is referred to as plasmolysis. This causes the protoplasm (all the material on the inside of the cell wall) to shrink away from the cell. If water is lost in excess, this can lead to the eventual collapse and death of the cell. As depicted in Figure 2, the protoplasm has visibly detached from the cell wall, and the cytoplasm is beginning to appear shriveled.
Another important concept that must be understood in order to analyze the observations found in this lab activity is water potential. Water potential can be defined as the potential energy of water in a system compared to pure water when both temperature and pressure are constant. To better understand water potential, it can also be defined as a measure of how freely water molecules can move in a particular environment (W5). The formula for water potential is (Ψ = pressure potential(ΨP) + solute potential(ΨS)).
Pressure potential is the pressure which develops in an osmotic system due to water entering or exiting the system (W13). In the case of this lab activity, the pressure potential is zero. Solute potential can be calculated by the formula ΨS= iCRT. The variable ‘i’ being the number of particles the molecule will make in water, ‘C’ being concentration, ‘R’ being the pressure constant 0.0831 liter bar/mole K, and ‘T’ being the temperature in Kelvin. Solute potential represents the component of water potential that is due to the presence of solute molecules (W7). In this experiment, the water potential of celery with each concentration will be measured and calculated using observations found during the procedure.
Last but not least, there are a few safety precautions that must be taken while completing this experiment. First, all beakers and dishes used should be washed with soap and water beforehand to ensure there is no chemical contamination from previous lab activities.
Additionally, the knife should be used cautiously when cutting small pieces of celery to avoid cutting the skin or fingers. Solutions and breakable materials should be transported with caution as to avoid breaking materials or spilling contents. Finally, hot plates should be monitored at all times and direct skin contact with boiling water should be avoided at all times.
Materials:
Lab A: Celery Passive Transport
- 6 Petri Dishes
- Ruler
- Knife
- 1 stick of celery
- The following concentrations of water; pure distilled water, 1.2g/100mL, 0.4g/100mL, 1.6g/100mL, 0.8g/100mL, and 2.0g/100mL(enough to completely engulf each piece of celery in the petri dishes)
- 6 pieces of paper
- Pen or pencil
- 500mL beaker
- A timer
Lab B: Yeast Active Transport
- Hot plate
- 2 test tubes
- 2 pieces of tape
- Pen or pencil
- Test tube stand
- 5mL of fresh yeast suspension
- Bottle of congo red dye
- Microscope
- 2 glass slides
- 2 droppers
- 1 500mL beaker
- 200mL of water
- Test tube tongs
Procedure:
- Complete the following for passive transport:
- Make the following solutions:
- pure distilled water b) 0.4g/100ml c) 0.8g/100ml d) 1.2g/100ml e) 1.6g/100ml f) 2.0g/100ml
- Label and fill 6 petri dishes with the above solutions.Remove the outer layer of a celery stalk (tough green tubes) and cut the white pulp that’s left into six rectangles 5mm thick and 30 mm long. Measure and record the length of the long side of each piece, about 30mm.Put a piece of cut stalk into each solution, be sure they are covered. After 35 minutes remove celery stalks and measure the long lengths again. Record observations from the class in a table including osmotic potential.
- Record the temperature of each solution.
- Graph the class ave. Δlength for each dilution and insert a line of best fit.
- Determine the osmotic potential of each solution using the instructions on the back. Assume that 1%= 0.01M
- Complete the following using living yeast cells:
- Label 2 test tubes: boiled and the other normal using a sharpie.Add 5mL of fresh yeast suspension to each test tube.Put the tube labeled “boiled” in a water bath and boil for 10 minutes. Remove using test tube tongs and let cool.Add 6 drops of Congo Red dye, shake and let stand for 5 minutes.
- Using a different eye dropper for each, observe under a microscope and make a proper labeled biological drawing of the boiled and unboiled yeast cells. Note the location of the dye.
Observations: Include a properly formatted table, graph and 2 diagrams of yeast.
Graph #1 – Difference in Length of Celery When Soaked in Various Concentrations of Salt This graph depicts the relationship between concentration of surroundings and the change in length of a piece of celery after being soaked in said solution.
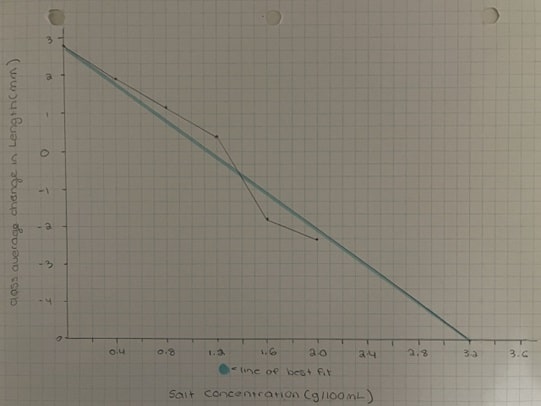
Table #1: Quantitative Analysis of Celery Experiment
This table contains the quantitative observations necessary for calculating the water potential of each piece of celery in the given concentration of salt water.
Concentration (g/100mL) | Length of long side before (mm) | Length of long side after (mm) | Difference in length (recorded from our experiment) (mm) | Difference in length (according to class average) (mm) |
Pure water | 33.0 | 35.0 | +2 | 2.8 |
0.4 | 20.0 | 31.0 | +2 | 1.9 |
0.8 | 25.0 | 27.0 | +2 | 1.3 |
1.2 | 30.0 | 34.0 | +4 | 0.4 |
1.6 | 32.0 | 36.0 | +4 | -1.7 |
2.0 | 30.0 | 31.0 | +1 | -2.3 |
Table #2 – Quantitative Change in Length Collected by Class at The End of Lab Experiment
This table demonstrates the varying range of data collected by each group in the class, providing the necessary information to determine an average change in length for each concentration.
Solution (g/100mL) | Change in Length (+/-mm) | |||||||||
+1 | +7 | +7 | +1 | +6 | +1 | +1 | +0 | +2 | +2 | |
0.4 | +7 | +3 | +2 | +1 | +2 | +4 | +1 | -1 | ||
0.8 | +1 | +2 | +6 | -2 | -2 | +3 | +1 | +4 | ||
1.2 | -1 | -2 | +1 | +4 | -3 | +1 | +1 | +3 | ||
1.6 | -1 | -3 | -1 | -4 | -1 | -2 | -3 | +2 | -3 | -1 |
2.0 | -2 | -3 | -5 | +1 | +1 | -5 | -3 | +3 | -5 | -5 |
Table #3 – Water Potential Relative to Change in Length and Concentration of Solution Celery is Immersed in
This table compares and contrasts the relationship between water potential, change in length, and concentration of salt water solution.
Concentration (g/100mL) | Change in Length (mm) | Water Potential |
2.8 | ||
0.4 | 1.9 | 0.19 |
0.8 | 1.3 | 0.39 |
1.2 | 0.4 | 0.58 |
1.6 | -1.7 | 0.78 |
2.0 | -2.3 | 0.97 |
Table #4: Qualitative Analysis of Celery Experiment
This table includes all images taken during the celery portion of the lab experiment as well as short descriptions of each image.
Image | Description |
![]() | This image pictures each of the pieces of celery minutes after being immersed in their designated solution with a label describing the corresponding solution. |
![]() | This image represents the piece of celery immersed in pure water after 35 minutes of soaking in the solution. |
![]() | This image represents the piece of celery immersed in 0.4g/100mL solution after 35 minutes of soaking in the solution. |
![]() | This image represents the piece of celery immersed in 0.8g/100mL solution after 35 minutes of soaking in the solution. |
![]() | This image represents the piece of celery immersed in 1.2g/100mL solution after 35 minutes of soaking in the solution. |
![]() | This image represents the piece of celery immersed in 1.6g/100mL solution after 35 minutes of soaking in the solution. |
![]() | This image represents the piece of celery immersed in 2.0g/100mL solution after 35 minutes of soaking in the solution. |
Table #5: Qualitative Analysis of Yeast Experiment
This table includes all images taken during the yeast portion of the lab experiment as well as short descriptions of each image.
Image | Description |
![]() | This image depicts the test tubes containing living yeast cells being boiled in a 500mL beaker over a hot plate. |
![]() | This image demonstrates the qualitative difference between the unboiled and boiled yeast cells without the use of a microscope. |
![]() | This image represents the test tubes containing boiled and unboiled yeast cells after adding congo red dye. |
![]() | Dyed unboiled yeast cells as seen through a microscope. |
![]() | Dyed boiled yeast cells as seen through a microscope. |
Diagrams 1 & 2
The following diagrams clarify the images of the boiled and unboiled yeast cells at a microscopic level.
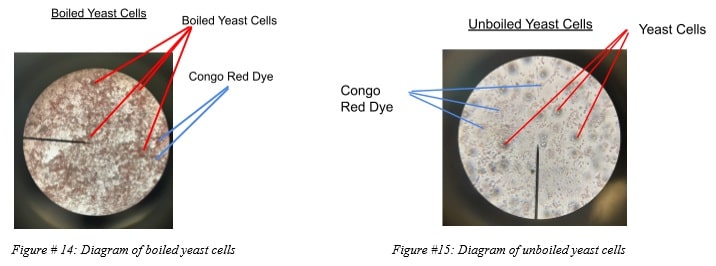
Calculations:
Calculation #1 – Change in Length of Celery Pieces in Pure Water ΔL = Change in length (mm) = ?
L2 = Final length (mm) = 35.0 L1 = Initial length (mm) 33.0 ΔL = L2 – L1
ΔL = 35.0mm – 33.0mm ΔL = 2.0mm
∴ According to the values taken from observations made (See Table 1), the change in length
when soaked in pure distilled water is 2.0mm.
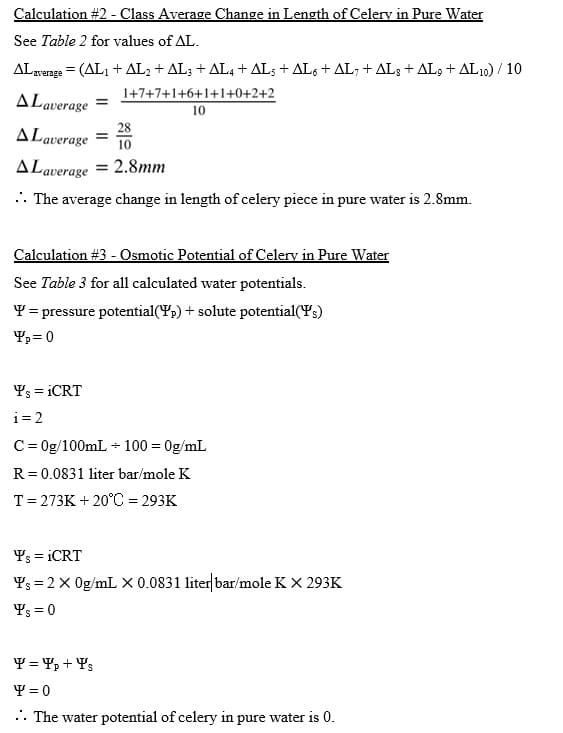
Conclusion:
The lab allowed the effects of various salt concentrations within solutions and how a second object or solution would react in regards to contact with one of these many different concentrations, and what effects can be observed in regards to osmosis, transport, and osmotic pressure to be studied and observed tremendously. Through the various combinations of various salt concentrations regarding its effects on celery, it’s logical to agree that the data demonstrated just how the various concentrations affected the celery and what it says about the isotonic salt concentration of celery.
Celery has an isotonic salt concentration of 1.0g/100mL; thus, when the celery was placed in solutions of higher salt concentrations like 1.6 and 2.0, there was a decrease in length of the celery due to the celery taking the role of the hypotonic solution in comparison to the salt solution playing the role of the hypertonic solution. The roles are then essentially in reverse when the concentration of the salt solution is lower than the celery’s 1.2g/100mL concentration.
As well as utilizing this lab experiment allows for observations to be made regarding how boiling one set of yeast cells can affect active transport when compared to a non-boiled sample of yeast cells. When observing the boiled sample of yeast cells, it is safe as well as logical to infer that during the process of boiling the yeast cells, the cells are reaching a higher temperature where it is much more difficult to survive unharmed or at all; this means that it can be inferred that the boiling of the yeast cells will affect the membranes ability to dictate what can and cannot diffuse through the membrane making it easier for the dye to diffuse into the individual cells.
This hypothesis was confirmed through the utilization of the microscope to observe changes in the cells microscopically to observe if the dye was able or unable to diffuse successfully into one sample or the other. Microscopically, it was observed that the non-boiled yeast cells were not only moving but did not appear to have diffused the red dye into the cells. In contrast, the boiled yeast cells were unmoving and were predominantly red, allowing for the inference that the red dye managed to seep through the seemingly damaged cell and its membrane.
A procedural issue to be noted within this experiment is the lack of specification regarding the appropriacy of placing each piece of the six celery into their solutions. This could lead to one piece being left in one solution longer than another, which can cause unfair and unequal balances within the amount of time each piece of celery spent in its own solution. Changing this specification can allow for more accurate, fair, and uncompromised data on the growth of the celery and its length.
An alternative procedural issue that emerged within this experiment was the lack of specification towards the truly considered quantity of a single drop from a dropper. This could lead to the under-dying of the yeast or over-dying of the yeast cells, which would highly alter and affect the quality of any observations and inferences about the lab and its results. This can be repaired through the specification of what quantity is to be considered a single drop out of six through the perspective of whoever created the experiment to ensure that this experiment and its results can be done again by another individual successfully.
Works Cited
(W1) Celery or Carrot in Salt Solution vs Distilled Water. Solutions 13.1-lecture demonstrations-department of chemistry-university of Massachusetts Amherst. (n.d.).
Retrieved March 6, 2023, from https://lecturedemos.chem.umass.edu/solutions13_1.html
(W2) Celery stalks that are immersed in fresh water for several hours become stiff and hard.
Study.com. (n.d.). Retrieved March 6, 2023, from https://homework.study.com/explanation/celery-stalks-that-are-immersed-in-fresh-water-f or-several-hours-become-stiff-and-hard-stalks-left-in-a-salt-solution-become-limp-and-so ft-from-this-we-can-deduce-that-the-cells-of-the-celery-stalks-are-a-hypotonic-to-both-fr esh-water-and-the-salt-sol.html#:~:text=Since%20water%20flows%20by%20osmosis,co ncentration%2C%20than%20the%20fresh%20water
(W3) Editors, B. D. (2019, October 5). Isotonic vs. hypotonic vs. Hypertonic Solution: Biology.
Biology Dictionary. Retrieved March 6, 2023, from https://biologydictionary.net/isotonic-vs-hypotonic-vs-hypertonic-solution/
(W4) Editors, B. D. (2019, March 29). Plasmolysis – definition, types and examples. Biology Dictionary. Retrieved March 6, 2023, from https://biologydictionary.net/plasmolysis/
(W5) Editors, B. D. (2017, April 29). Water potential – definition, Formula & Quiz. Biology Dictionary. Retrieved March 6, 2023, from https://biologydictionary.net/water-potential/
(W6) Elsevier Current Trends. (2009, March 18). Vesicular transport across the fungal cell wall.
Trends in Microbiology. Retrieved March 6, 2023, from https://www.sciencedirect.com/science/article/abs/pii/S0966842X09000420#:~:text=Rece nt%20findings%20indicate%20that%20fungi,originate%20from%20cytoplasmic%20mul tivesicular%20bodies
(W7) Encyclopedia.com. (2023, March 7). .” A dictionary of biology. . encyclopedia.com. 22 Feb. 2023 . Encyclopedia.com. Retrieved March 6, 2023, from
https://www.encyclopedia.com/science/dictionaries-thesauruses-pictures-and-press-releas es/solute-potential-1
(W8) Explain plasmolysis in a plant cell. draw a diagram showing stages in … (n.d.). Retrieved March 7, 2023, from
https://www.toppr.com/ask/question/explain-plasmolysis-in-a-plant-cell-draw-a-diagram- showing-stages-in-plasmolysis/
(W9) Passive Transport. FutureLearn. (n.d.). Retrieved March 6, 2023, from https://www.futurelearn.com/info/courses/introduction-to-human-cells-the-basis-of-life/0/ steps/330524
(W10) JS;, C. (n.d.). Properties and metabolism of the aqueous cytoplasm and its boundaries.
The American journal of physiology. Retrieved March 6, 2023, from https://pubmed.ncbi.nlm.nih.gov/6364846/#:~:text=The%20nucleoplasm%2C%20the%2 0interiors%20of,aqueous%20compartments%20of%20animal%20cells
(W11) Turgor pressure. Biology Articles, Tutorials & Dictionary Online. (2022, June 16).
Retrieved March 6, 2023, from https://www.biologyonline.com/dictionary/turgor-pressure
(W12) Water in plants. Biology Articles, Tutorials & Dictionary Online. (2020, October 20).
Retrieved March 6, 2023, from
https://www.biologyonline.com/tutorials/water-in-plants#:~:text=Water%20enters%20pla nt%20cells%20from,and%20the%20plant%20will%20die
(W13) Water potential | definition, examples, diagrams – TOPPR ASK. (n.d.). Retrieved March 7, 2023, from
https://www.toppr.com/ask/content/concept/water-potential-solute-potential-and-pressure-potential-201323/
(W14) What is osmosis? FutureLearn. (n.d.). Retrieved March 6, 2023, from https://www.futurelearn.com/info/courses/teaching-biology-inspiring-students-with-plant s-in-science/0/steps/58750
(W15) What temperature kills yeast. Bob’s Red Mill Blog. (2018, February 21). Retrieved March 6, 2023, from
https://www.bobsredmill.com/blog/baking-101/what-temperature-kills-yeast/#:~:text=To o%20Hot%20to%20Survive,will%20be%20completely%20killed%20off